Vehicle Dynamics: The Basics You Need to Know
Introduction
Vehicle dynamics is the study of how vehicles move and the forces that cause these movements. It looks at how a vehicle responds to different factors like driver inputs, external forces, and environmental conditions. Knowing how vehicle dynamics work is essential for anyone who wants to drive better. Whether you’re just starting out or have been driving for years, understanding these concepts can help you improve your skills and performance. In this article, we’ll break down the basics of vehicle dynamics in a way that’s easy to grasp.
Table of contents
- Main Targets
- The Principles of Vehicle Dynamics
- Understanding the Vehicle Axis System
- Vehicle Basic Dimensions
- Forces acting on a racecar
- Conclusion
Main Targets
The primary goal is to grasp the principles of vehicle dynamics to enhance a vehicle’s performance. By understanding how different forces and inputs affect a car’s movement, we can make informed adjustments that boost efficiency, safety, and overall driving experience. This knowledge is key to getting the most out of any vehicle, whether you’re on a race track or navigating everyday roads.
The Principles of Vehicle Dynamics
The primary goal is to grasp the principles of vehicle dynamics to enhance a vehicle’s performance. By understanding how different forces and inputs affect a car’s movement, we can make informed adjustments that boost efficiency, safety, and overall driving experience. This knowledge is key to getting the most out of any vehicle, whether you’re on a race track or navigating everyday roads. Let’s explore these dynamics in detail to unlock your vehicle’s full potential.
Understanding the Vehicle Axis System
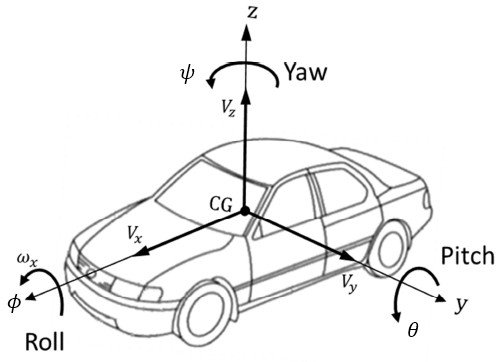
The image above illustrates the vehicle axis system, which is essential for understanding how a vehicle moves and responds to various forces. The system includes three main axes: longitudinal (x), lateral (y), and vertical (z). Each axis is associated with specific types of motion:
- Roll (φ or ωx): This refers to the tilting motion of the vehicle around the longitudinal axis (x-axis). Roll typically occurs when the vehicle takes a turn, causing the body to tilt to the outside of the curve due to centrifugal force.
- Pitch (θ or ωy): This is the tipping motion around the lateral axis (y-axis). Pitch happens when the vehicle accelerates or decelerates, causing the front or rear to lift or dip.
- Yaw (ψ or ωz): Yaw describes the rotation around the vertical axis (z-axis). It is the turning motion of the vehicle, such as when steering the car left or right.
The labels Vx, Vy, and Vz represent the vehicle’s velocity components along the x, y, and z axes, respectively. The center of gravity (CG) is the point where the vehicle’s mass is considered to be concentrated, and it plays a crucial role in how these dynamics affect the vehicle’s stability and handling.
By understanding these axes and motions, drivers can better comprehend how their actions, like steering, braking, and accelerating, impact the vehicle’s behavior. This knowledge allows for more precise control and improved performance, making driving not only more efficient but also safer.
Vehicle Basic Dimensions
The image above illustrates the basic dimensions of a vehicle, which are crucial for understanding vehicle dynamics. These dimensions influence how a vehicle handles, its stability, and overall performance. Here’s a breakdown of the key terms shown in the image:
- L (Wheelbase): This is the distance between the center of the rear wheel and the center of the front wheel. A longer wheelbase generally provides better stability at high speeds, while a shorter wheelbase can enhance maneuverability and responsiveness.
- h (Height of the Center of Gravity): This measures how high the vehicle’s center of gravity is from the ground. A lower center of gravity improves stability and reduces the risk of rollover, especially during sharp turns or sudden maneuvers.
- b (Front Overhang): This is the longitudinal distance from the center of gravity to the center of the front wheel. It affects the vehicle’s weight distribution and handling characteristics.
- c (Rear Overhang): This is the longitudinal distance from the center of gravity to the center of the rear wheel. Like the front overhang, it influences the vehicle’s balance and handling.
Understanding these dimensions helps in assessing a vehicle’s design and predicting its behavior under different driving conditions. For instance, vehicles with a lower center of gravity and balanced overhangs are typically more stable and easier to control. These measurements are fundamental for engineers when designing vehicles for optimal performance and safety.
The image above illustrates the concept of track width, which is a critical parameter in vehicle dynamics. Track width refers to the distance between the centers of the tires on the same axle. The diagram shows two different vehicles with their respective track widths labeled as ttt_ttt (track width for the first vehicle) and tdt_dtd (track width for the second vehicle).
- Track Width (tt and td): Track width is the horizontal distance between the centerlines of the wheels on the same axle. A wider track width generally provides better lateral stability and reduces the likelihood of rollover, especially during sharp turns. Conversely, a narrower track width can enhance the maneuverability of the vehicle but may compromise stability.
Key Implications of Track Width:
- Stability: A wider track width lowers the vehicle’s center of gravity and improves stability, making it less prone to tipping over during cornering.
- Handling: Wider track widths can improve handling by allowing for better distribution of lateral forces during turns. This can result in a more predictable and controlled driving experience.
- Performance: The optimal track width can vary depending on the type of vehicle and its intended use. Sports cars often have wider track widths to enhance handling and performance, while off-road vehicles may have narrower tracks to navigate rough terrains more effectively.
The image above illustrates the Ackermann steering principle, which is crucial for understanding vehicle steering geometry and its effects on handling. This principle ensures that all four wheels of a vehicle follow concentric circles when the vehicle is turning, preventing tire scrubbing and improving handling.
Key Components and Concepts:
- Steering Angles (δo and δi): The angles at which the outer (δo) and inner (δi) wheels turn. In a turn, the inner wheel must turn more sharply than the outer wheel to maintain proper alignment with the turn center.
- Wheelbase (L): The distance between the front and rear axles of the vehicle. A longer wheelbase generally contributes to smoother handling but may reduce maneuverability.
- Track Width (t): The distance between the left and right wheels on the same axle. A wider track width can enhance stability during turns.
- Turning Radius (R): The radius of the circle that the vehicle’s path describes when making a turn. This is influenced by the steering angles and wheelbase.
Forces acting on a racecar
Understanding the fundamental forces acting on a vehicle is crucial for mastering vehicle dynamics. The image above highlights these essential forces and provides the formulas to quantify them.
-
Weight (Gravitational Force):
- Formula: W=m⋅g
- Where m is the mass of the vehicle and g is the acceleration due to gravity. This force pulls the vehicle towards the ground, affecting traction and stability.
-
Aerodynamics:
- Drag Force: This force opposes the vehicle’s motion through the air.
- Formula: Fdrag=1/2⋅ρ⋅A⋅Cd⋅v²
- Where ρ is the air density, A is the frontal area of the vehicle, Cd is the drag coefficient, and v is the vehicle’s velocity.
- Downforce: This force pushes the vehicle down onto the road, increasing traction.
- Formula: FDF=1/2⋅ρ⋅A⋅Cz⋅v²
- Similar to the drag formula, but Cz is the lift coefficient.
- Drag Force: This force opposes the vehicle’s motion through the air.
-
Road-Tyre Vertical Forces: These are the forces exerted by the road on the tires vertically, supporting the vehicle’s weight and contributing to traction.
-
Road-Tyre Friction Forces: These forces are crucial for acceleration, braking, and cornering, as they provide the necessary grip between the tires and the road surface.
-
Inertial Forces:
- Centripetal Force: This force acts towards the center of a curve when the vehicle is turning, keeping it on its curved path.
- Formula: Fcentripetal=m⋅v²/r
- Where m is the mass of the vehicle, v is the velocity, and r is the radius of the turn.
- Centrifugal Force: This is the outward force experienced by the vehicle during a turn, opposing centripetal force.
- Centripetal Force: This force acts towards the center of a curve when the vehicle is turning, keeping it on its curved path.
-
Longitudinal Forces:
- Braking Force: The force applied by the brakes to slow down the vehicle. This force acts opposite to the direction of motion and is crucial for vehicle control.
- Traction Force: The force exerted by the engine through the drive wheels to propel the vehicle forward. This force must overcome both rolling resistance and drag to accelerate the vehicle.
-
Lateral Forces:
- Cornering Force: The lateral force generated by the tires during a turn, which helps the vehicle to navigate curves. This force depends on the tire’s grip and the vehicle’s speed.
-
Suspension Forces:
- Spring Force: The force exerted by the suspension springs, which absorb shocks from the road and maintain tire contact.
- Damping Force: The force exerted by the shock absorbers, which control the rate of spring compression and extension, reducing oscillations and improving ride quality.
-
Rolling Resistance:
- This is the resistive force that opposes the motion of the vehicle due to the deformation of the tires and the road surface.
- Formula: Frolling=Cr⋅N
- Where Cr is the rolling resistance coefficient and N is the normal force (vehicle weight).
Conclusion
Understanding the various forces acting on a vehicle is fundamental to mastering vehicle dynamics. These forces—ranging from gravitational and aerodynamic forces to road-tyre friction and suspension dynamics—play a critical role in determining how a vehicle performs and handles in different driving conditions. By comprehending and optimizing these forces, drivers and engineers can significantly enhance vehicle stability, control, and efficiency.
This guide offers a basic overview of vehicle dynamics. For those looking to dive deeper into the intricacies of vehicle behavior and performance, stay tuned to our website. We will be providing advanced posts that cover more detailed and technical aspects of vehicle dynamics. Whether you’re an automotive engineer, a racing enthusiast, or simply a curious driver, our upcoming content will equip you with the knowledge to push the boundaries of vehicle performance.